New research suggests what will make us smarter, thinner, and happier. In most cases, timing is everything.
by Eric Celeste | Illustration by Tom Haugomat
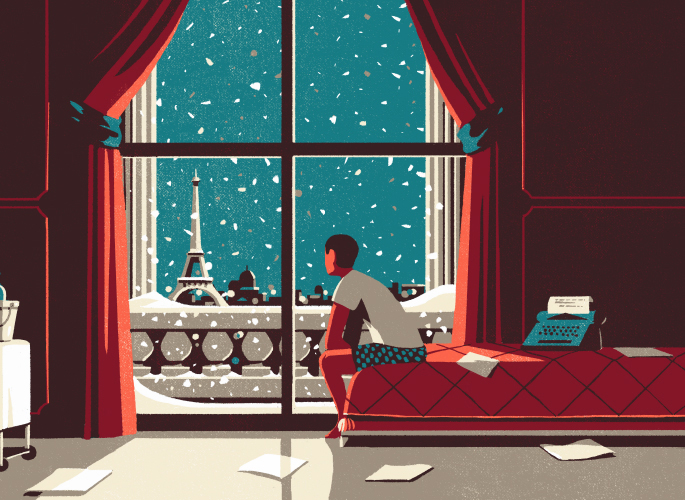
How do you make a discovery when you know nothing about what you’re studying? When you’re not even sure what you’re looking for? Where do you start?
Those were the crucial, career-defining questions that Dr. Joseph Takahashi and his team faced nearly a quarter-century ago. It was the early 1990s, and Takahashi — now the Chair of the Department of Neuroscience and an Investigator in the Howard Hughes Medical Institute at the University of Texas Southwestern Medical Center — was just entering the high-profile phase of his professional journey, one in which he sought to better understand our bodies’ complex behaviors through gene discovery. His goal was to uncover the genetic secrets of our circadian rhythms — what most people call our body clock.
The human body has a master clock in the brain, but every cell has its own clock, now thought to regulate multiple functions that determine the quality of our days. By unlocking these genetic codes, we have found answers to questions like how to sleep through the night; how to eat without gaining weight; even what time of day we should have surgery or undergo chemotherapy. In short, they’re the genetic answers to how we can live our best lives.
That Takahashi would find this as his lifelong work was serendipitous. He was born in Tokyo. His father was Japanese-American and worked for Gen. Douglas MacArthur in the U.S. Army in postwar Japan; there he met Takahashi’s mother, a Japanese woman of aristocratic descent. Takahashi spent much of his life traveling the world with his family, as his father would take consulting jobs in economics around the world. As a boy, Takahashi was a natural explorer, fascinated with systems both animal (the elephants of Burma, now Myanmar; the camels of Pakistan) and mechanical (the motorcycles and cars of Rome).
Takahashi finished high school in America, and almost as soon as he got to college, he began devising experiments that would help to set his path as a researcher.
“In college, I took this course on the physiological basis of animal behavior,” Takahashi recalls. “The professor, Kenneth Rawson, had a lab exercise on electric fish, and we’d measure their discharge. I was fascinated by that, just because I’d never seen these unusual fish before, and that they would actually send off these electrical discharges.”
Takahashi asked if he could keep recording from a particular fish. He and the professor set up a little chart recorder. “What we found is that if you didn’t disturb this fish in the daytime, it went totally silent. And then it only fired at night, when it was swimming around. It turned out to be nocturnal. Once we figured that out, then we decided to do systematic experiments.”
Takahashi found other fish that followed the first one’s patterns: They went totally silent while resting, then fired their idiosyncratic electrical discharges when active. “It was circadian,” Takahashi realized. “That got me started.”
By 1997, they’d cloned the clock gene, becoming the first scientists to successfully clone a circadian clock gene in a mammal.
Now, more than four decades later, his study of circadian clocks has revealed their powerful influence over many of the most basic aspects of our lives.
Takahashi, who worked in Northwestern University’s departments of neurobiology and physiology for 26 years before coming to UT Southwestern in 2009, decided that he and his lab would take the scientific road less traveled.
Their approach would be random, unbiased — and exceedingly difficult. Instead of looking at a specific gene and figuring out what it might do to a person, they worked the other way — looking at the outcome of a genetic mutation and deducing which gene mutation caused it. This model of discovery is called forward genetics, and it’s best known as the method that led to the discovery of the gene for Duchenne muscular dystrophy and, later, the gene for cystic fibrosis.
If Takahashi’s methodological choice seems unusual, then his next decision seems positively insane, scientifically speaking. He settled on mice as his research subjects. Mice are more expensive than what most scientists use for similar studies — fruit flies and worms — and breed far less often than these “model organisms.” It could have taken tens of years and thousands of mice before Takahashi found one that could lead his team to a breakthrough.
It was highly unlikely that they’d find it in the first batch of mice they studied. But that was what happened. Their breakthrough mouse came to be known as No. 25, the 25th mouse in the batch. Once No. 25 produced grandchildren, Takahashi and his team generated charts of the offspring running on wheels in total darkness that showed they’d mutated to have a 28-hour circadian clock. Takahashi knew that was a big day. “Whoa,” he says now, recalling his reaction, “this is amazing.”
Now the process could begin, a process that was “like a treasure hunt,” Takahashi says now. They’d found the mutated pattern. Next they had to find the gene that caused the mutation. It took 10 people working three years — 30 years of lab time — but in 1994, Takahashi and his team had narrowed down the gene to a chromosome; and by 1997, they’d cloned the clock gene, becoming the first scientists to successfully clone a circadian clock gene in a mammal. That opened up a number of doors to understanding the function of the gene in humans, and how our body clocks regulate our lives.
“Cloning a gene is like this restriction point that we have to get through, that’s holding us up,” says Takahashi, a member of the National Academy of Sciences and the National Academy of Medicine. “Once we get through this thing, everything opens up on the other side, because once you find that gene, you can find the next gene, and then you can try to understand what that gene is doing.”
"Once you find that gene, you can find the next gene, and then you can try to understand what that gene is doing."
Takahashi’s discoveries have been groundbreaking not just for science but in their applicability to everyday lives. From his research, Takahashi has made, and helped lead others to, enough discoveries about complex human processes to fill the pages of a modern self-help book. This is what it looks like.
Sleeping
Our circadian clocks control the timing of sleep — when we sleep, and the amount we sleep. “They help determine whether we’re early birds or night owls,” Takahashi says. “Before, people would think that those were just personality traits, but in reality we know that they are actually genetically determined. Not in a simple way; it’s usually not just one gene. We have a whole collection of genes that are going to genetically program you to be either an owl or a lark.” This is probably why, he notes, we self-select for the types of jobs that best fit with our clocks — shift work, for example. Our clocks are unique among animals in that we sleep for large consolidated blocks of time, seven to nine hours at a time. And our modern lifestyle and work schedules tend to be misaligned with our internal clocks, resulting in a kind of “social jet lag”: Our workweek deprives us of needed sleep, and our clocks work overtime on weekends to make up or bank that sleep before the cycle begins anew.
Eating
Takahashi says that a current diet trend known as “intermittent fasting” — the idea that we should only eat during a defined period during the day, e.g., eight-hour windows, and fast for the remaining 16 hours — marries well with the research he’s working on. “If you give a mouse a high-fat diet,” he says, “it becomes obese. But if you restrict when it can eat the food to only the proper time, which is the nighttime for a mouse ... the mouse doesn’t get fat. Same diet. If you give it that diet in the daytime, when it’s not supposed to eat, it becomes obese.” Takahashi notes that he isn’t always good at sticking to this plan himself, primarily because a glass of after-dinner wine often falls outside the ideal circadian eating window.
Metabolism
“Metabolism is huge,” Takahashi says. “We can show there are literally thousands of genes that our clock controls that are involved in metabolism.” He points to one example: the liver. “In your liver, which is a major metabolic organ, and also your muscles and your fat — those are all metabolic tissues — the clock is controlling thousands of genes in all of these tissues and turning them on and off each day. So our entire metabolism is on a 24-hour program.” When the clock gene is turned on (in daylight hours, for humans), our metabolism is faster. When it’s turned off, our metabolism is slower. That’s partly why it’s so important to stick to the optimal eating window.
Disease Treatment
“We know, we’ve actually known for decades, that cancer therapy has higher rates of morbidity depending on the time of day,” Takahashi says. “Give the mouse a dose at one time of day, and the mouse will tolerate it. At the opposite time of day, it’ll kill the mouse. So that kind of toxicity is extremely well-established. We even showed that in our own experiments. So that’s one effect. It’s a side effect or worse at one time of day compared to the other for different chemotherapies. Is there going to be differential efficacy at different times? Those kinds of trials are being done in Europe.”
Takahashi laments that scientists are having trouble establishing such breakthroughs in disease-focused labs in the U.S. You feel as though he’d like to reach out and tell the labs that the wonders of our internal clocks know few bounds. That it’s OK to look at a complex system of research even if you’re not sure where it will lead. That sometimes it’s OK to say, “Where do you start?” With a little luck, the answers keep coming for decades.
Takahashi’s discoveries have been groundbreaking not just for science but in their applicability to everyday lives.
Setting Your Clock
A day in your life, when you live by your circadian clock.
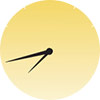
7:42 A.M. – Wake up
You don’t set an alarm because you’re in tune with your system, which normally wakes you slowly, peacefully, before 8 a.m. — plenty of time to get your day started because work schedules better conform to sleep schedules in our idealized future.
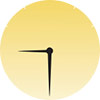
9:30 A.M. – Head to airport
You are on your way to catch your 11 a.m. flight to Boston. Because of studies done on the effects of jet lag on baseball players, you know the downside of taking flights too early or late in the day.
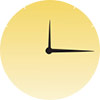
12:15 P.M. – Eat lunch
You’ve had coffee and club soda to keep you sated, but you take your first meal only when you’re hungry, and within the nine-hour window that ends when you expect to be done with your (late) dinner date. This more naturally aligns with your circadian system, and helps keep you from gaining weight.
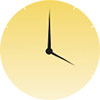
4:00 P.M. – Finish office work
Complete your videoconference meeting, and send in the work you’ve finished at the Boston-area coffee shop. You might be tempted to have another coffee to keep from crashing, but caffeine lingers in your body for a long time, so it’s better not to consume it late in the day.
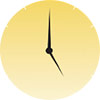
5:00 P.M. – Work out at hotel gym
You’re a night owl, and your doctor has determined it’s OK for you to get your exercise later in the day, since you’re always up until 11 p.m. anyway.
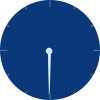
6:30 P.M. – Attend mother's chemotherapy treatment
Testing has shown your mom responds best to treatment in the early evening, so that’s when it’s scheduled. She’s been showing good signs, and is recovering well.
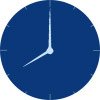
8:00 P.M. – Eat dinner at your hotel
You’re meeting a client, and knowing that you’d be eating late, you planned your ideal caloric-intake window.
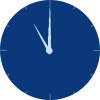
11:00 P.M. – Go to sleep
No matter the demands of your busy lifestyle, you need those eight-plus hours of sleep. You know, because your doctor told you, that recent studies show that sleep deprivation causes your leptin levels to go down, so then you’re hungrier and you eat more. And your next meal isn’t scheduled until midday tomorrow.